Modeling a Concentrated Solar Thermal Collector for Methane Dry Reforming
Hydrogen has great potential to be the fuel of the future through utilization in fuel cells, but it is rarely found naturally and must be produced in energy-intensive processes. Steam reforming, the process of heating natural gas and steam in the presence of a catalyst to drive a chemical reaction that produces hydrogen, currently accounts for 95% of hydrogen production. The heat for the reaction is typically provided by natural gas combustion, but using solar energy for heat is more sustainable. Concentrated solar power (CSP) is one promising method to harness solar energy more effectively by concentrating incident sunlight. The design consists of a vacuum-insulated collector with fluid flowing between two flat plates, and a Fresnel lens above to concentrate sunlight. The fluid is modeled as a water-methane mixture, with phase change occurring from water to steam. Accurately predicting temperatures and efficiency is critical to determining operational parameters for such a collector. The unique combination of phase change and fluid flow creates a modeling challenge for COMSOL, especially in 3 dimensions. To reduce computational time, an equivalent 2D model was created. 2 separate, connected collectors are considered, one non-concentrated collector for phase change from water to steam and one concentrated collector for superheating the mixture and allowing reaction. This model includes the Heat Transfer Module, Chemical Reaction Engineering Module, and 2 Multiphysics: Nonisothermal Flow to couple Heat Transfer and Laminar Flow, and Reacting Flow to couple Laminar Flow and Transport of Concentrated Species. The 2D model includes 2 copper flat plates with fluid flow between them, a glass cover to let light pass through, and mirrored surfaces on all other sides. The model includes surface-to-surface radiation with diffuse surfaces of various emissivities, split into solar and ambient values. The results show S-shaped curves for outlet temperature and methane conversion and an inverted S-shaped curve for efficiency, all vs. mass flowrate. The physical accuracy of the model is confirmed using mass and energy balances on the system. Using these results, we can identify the most efficient operating point to achieve the temperature required for reaction and hydrogen production. We can also determine the concentration necessary, and the optical properties required from the absorption coating at the required temperature. Further work includes comparison of this finite-element based method to an analytical solution, and the drawbacks/advantages of each method. Additionally, the results from the model will be compared to actual experimental data obtained from reacting steam with methane in a catalyst placed in a tube furnace operating at various temperatures, creating similar processes as the model. This allows real-world verification of the model’s results.
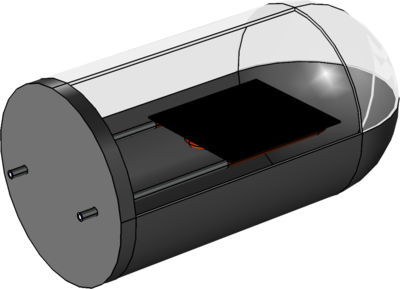
下载
- verma_poster.pdf - 0.65MB
- verma_paper.pdf - 0.43MB
- verma_abstract.pdf - 0.02MB