Thermal Model and Control of Metal-Organic Chemical Vapor Deposition Process
Metal-Organic Chemical Vapor Deposition (MOCVD) is used for the manufacture of Multi-Quantum Well Light Emitting Diodes (MQW LEDs). The process uses a carrier gas flow containing a dilute mixture of metal organic precursors, Tri-Methyl Gallium (TMG) and Tri-Methyl Indium (TMI), to epitaxially deposit GaN and InGaN on a heated wafer of diameter ranging from 2" to 8". The gas mixture flows into a reactor chamber at 50-500 torr where substrates are heated to about 800°C for depositing InGaN and other conventional III-V materials, and as high as 1200°C for GaN. The reactive gases decompose and deposit thin epitaxial layers whose thicknesses range from a few nanometers to a few microns thick as required. Byproducts such as methane are swept out of the chamber. During process, the precursors are changed depending on the layer being deposited, and the substrate temperature switches periodically between 800°C and 1200°C.
Variations in the deposition processes have significant impact on the color, lumens, and forward voltage of the LEDs. Even after significant R&D efforts in the industry to minimize this production variation, the current technology is not capable of producing LEDs that are highly consistent and tightly controlled with respect to the above three quantities. To address this problem, LED manufacturers group the devices into "bins" with each bin spanning a range of color temperature, voltage or lumens. The larger the bin size, the greater the variation in light color or output – smaller bins have tighter control.
The color of the light emitted by an LED is a strong function of the wafer temperature during the deposition process. Hence, accurate temperature control of the MOCVD process is essential for making the bins smaller, and eventually eliminating them. For MOCVD, the wafers are placed in pockets in a graphite susceptor that is heated from below. The thermal contact resistance between the wafer and the susceptor results in a temperature drop from the susceptor to the wafer. Since the wafer is cooling to the top of the chamber, it will typically bow up (edge high) resulting in a radial variation in this contact resistance, which, in turn, results in a temperature variation across each wafer. Such temperature nonuniformity across the wafer is the primary cause of LED performance variations resulting in the need for binning. Only improved temperature control can mitigate this "binning" problem.
SC's proven model-based control technology addresses this problem starting with the development of a thermal model of the reactor that accurately predicts the temperature distribution of the susceptor and wafers. To this end, SC is collaborating with RPI in developing a model of their Thomas Swan showerhead reactor whose susceptor accommodates three wafers each with 2" diameter (3×2 reactor). We have developed a 3D model of RPI's MOCVD reactor using COMSOL Multiphysics, and compared the results by conducting an experimental study. We will present results of model calibration and validation with process data. A reduced-order version of the model will be used to develop an effective control strategy.
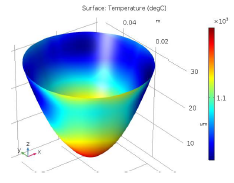
下载
- ebert_presentation.pdf - 1.46MB
- ebert_poster.pdf - 0.5MB
- ebert_abstract.pdf - 0.02MB