Electrothermal Actuation of Nanoscale Transducers: Modeling and Experimental Validation
Electrothermal actuators convert an electrical signal into a mechanical signal via Joule heating. Due to their simplicity and reliability, electrothermal actuators are commonly used in various fields across length scales: from human-scale soft robotics to nanometer-scale sensors. We investigate electrothermal actuation of nanomechanical motion using a combination of COMSOL numerical simulations and analytical solutions. Our nanoelectrothermal actuator structure is a u-shaped gold nanoresistor that is patterned on the anchor of a doubly clamped nanomechanical beam or a microcantilever resonator. A nanomechanical beam integrated with an electrothermal actuator forms a nanoelectromechanical systems (NEMS) device. This NEMS design has been used in recent experiments successfully, with applications in virus detection, mechanical computing, and gas sensing, among others. Thus, understanding the physics behind electrothermal actuation and transduction in nanoscale systems is vital for pushing the limits of nanomechanical sensing and nanotechnology. In our finite-element analysis (FEA) based model, our input is an ac current; we first calculate the temperature oscillations due to Joule heating using Ohm's Law and the heat equation; we then determine the thermally induced bending moment and the displacement profile of the beam by coupling the temperature field to Euler-Bernoulli beam theory with tension. Our COMSOL model efficiently combines transient and frequency-domain analyses: we compute the temperature field using a transient approach and then impose this temperature field as a harmonic perturbation for determining the mechanical response in the frequency domain. This unique modeling method offers lower computational complexity and improved accuracy, and is faster than a fully transient FEA approach. Our dynamical model computes the temperature and displacement fields in time domain over a broad range of actuation frequencies and amplitudes. We validate the numerical results by directly comparing them with experimentally measured displacement amplitudes of NEMS beams around their eigenmodes in vacuum. Our model predicts a thermal time constant of 1.9 ns in vacuum for our particular structures, indicating that electrothermal actuation is efficient up to 80 MHz. We also investigate the thermal response of the actuator when immersed in a variety of fluids.
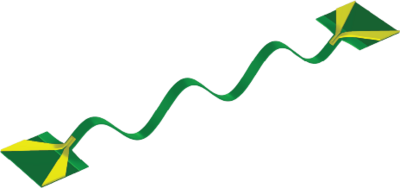