Mechanical Characterization of Skin Undergoing Large Deformation During Suction Loading
Skin, the largest organ in the body, comprises three major layers: the epidermis, dermis, and adipose tissue. Its complex microstructure results in non-linear stress-strain responses when subjected to large deformations, necessitating non-linear constitutive relations like the Mooney Rivlin and Ogden models to reproduce these responses accurately. Modeling the mechanical responses is crucial for developing medical devices that apply controlled external loading to the skin, like suction pressure. However, existing models for studying responses under suction pressure are predominantly 2D and for small deformations.
To address this gap, we developed a 3D computational model to investigate the mechanical properties of skin and adipose tissue under suction pressure that results in large deformations. This work aims to numerically evaluate the changes in tissue mechanical properties based on varying suction pressures.
Using COMSOL Multiphysics software, a 3D, steady-state, multi-layered, and symmetric model was developed to determine the bulk mechanical properties of each tissue layer by employing an inverse method. The thickness of the adipose layer was based on ultrasound images obtained from the volar forearm (5 mm). An average skin thickness of 2 mm was used. The Ogden model was used for the skin, and the Mooney-Rivlin model was used for the adipose tissue. A boundary condition representing uniform pressure up to 8 inHg was applied within a 50 mm diameter area. The circular cross-section resulted in a dome-shaped deformation. Experimental validation was conducted using a camera to record deformation. The maximum skin deformation (dome apex height) was measured. The hyperelastic constants for both layers were determined by minimizing the error in predicted deformation using the linear least squares method. The variability of the hyperelastic constants was then compared at different pressures.
At eight inHg, the dome apex height was 9.6 mm. The Ogden shear modulus in the forearm increased as pressure increased with an average value of 47.7 kPa and standard deviation of 3.06 kPa. Similarly, the Ogden strain hardening coefficient increased for the forearm with an average value of 25.263 and standard deviation of 1.17. The derived Mooney-Rivlin parameters at eight inHg were determined to be 2452.3 Pa and 2228.6 Pa. Utilizing the hyperelastic constants determined at eight inHg allowed for accurate prediction of displacement under other loading conditions, with a maximum error ranging between 2.6% and 10.3%. The magnitude of error decreased as loading pressure approached eight inHg.
The model enabled the determination of Mooney Rivlin and Ogden parameters as a function of external loading. Notably, under variable external loading, the skin's shear modulus exhibited greater variability compared to the skin's strain hardening coefficient, which remained relatively constant. However, for simulations employing these parameters, closer adherence to the loading pressure utilized for optimization is imperative to enhance accuracy. The 3D model provides valuable insights into tissue biomechanics and can facilitate medical device modifications.
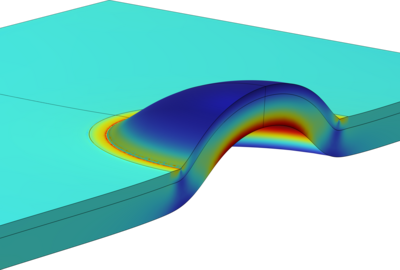