Modeling the Distribution of Active Phases in Alkaline Zn Anodes Using COMSOL
The discharge of an alkaline Zn anode involves the conversion of Zn metal to solid ZnO through the zincate ion. With the heterogeneous dissolution of Zn and mobility of zincate in the electrolyte, complex radial distributions of Zn and ZnO have been observed to occur in the anodes of commercial bobbin-type alkaline batteries. These distributions are highly dependent on the discharge rate and lead to phase distributions that are difficult to predict. The distribution of Zn and ZnO within these electrodes is relevant to battery performance, as localized pore closure and breakdown of the electronically conductive network can lead to premature cell failure. By quantitatively analyzing synchrotron tomography, in situ radial distributions of the active materials can be obtained under a range of discharge conditions and used to improve model accuracy. The CT results obtained for high-rate continuous discharge were in good agreement with conventional battery model predictions implemented in COMSOL. This showed localized discharge near the anode-separator interface. However, the results when using a low-rate continuous discharge protocol were vastly different and were not predicted by current models. In these cells, the discharge reaction inverted, showing substantial Zn dissolution and ZnO precipitation near the center current collecting pin. To better match predicted Zn and ZnO distributions to results measured by CT at low rates, we added two new physical phenomena to a conventional computational model, based on previous work from Mao and White.[1] This model was built in COMSOL Multiphysics with the Battery Design Module. Based on the quantitative results of CT experiments, it is very likely that under low-rate discharge conditions the electronic conduction network begins to break down as the cell discharges. To capture this phenomenon in the model, the anode’s electronic conductivity was changed from a Bruggeman-like relationship to one that takes percolation theory into account.[2] Additionally, CT results show substantial movement of the Zn particles within the battery during discharge. This was hypothesized to be due to volume expansion from the formation of ZnO at the interior of the anode. Further modification of the model was made to incorporate the granular flow of the Zn and ZnO phases to more accurately match the phase distributions observed by CT experiments during low-rate discharge. By incorporating percolation theory and granular flow of the solid Zn and ZnO phases, the model was able to accurately predict the phase distributions of the anode under low-rate discharge conditions.
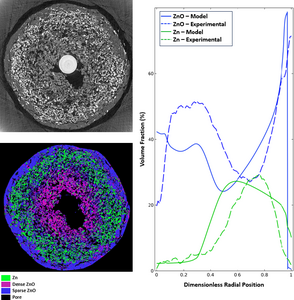